Pressure vessel:
A pressure vessel is a container or device designed to hold gasses or liquids at a pressure significantly different from the ambient pressure. These pressure vessels safely confine substances, preventing leaks or ruptures even in high-pressure conditions. Industries like textile chemical processing, petrochemical, and nuclear rely on them for pressure control. The design, construction, and operation of pressure vessels follow specific codes and standards to ensure safety and functionality. Here are the names of a few organizations related to pressure vessels:

Organizations related to pressure vessels:
Here are the names of a few organizations related to pressure vessels:
1. American Society of Mechanical Engineers (ASME):
ASME is a well-known organization that provides guidance and standards regarding pressure vessels and their usage.
2. American Society for Testing and Materials (ASTM International):
ASTM International establishes standards for testing and measurement related to pressure vessels and external pressure.
3. National Board of Boiler and Pressure Vessel Inspectors (NBBI):
NBBI sets standards and conducts inspections for pressure vessels and boilers, providing licenses for inspections of these entities.Other more regional organizations, in addition to the three mentioned above, set guidelines for the design, maintenance, and testing of pressure vessels.
Types Of Pressure vessel:
The three most popular types of pressure vessels are process vessels, heat exchangers, and storage vessels.
1. Storage Containers:
Proper storage demands pressurized containment for various products. Storage pressure vessels fulfill this need, each specially crafted to contain specific products at precise temperatures. Products like propane, ammonia, butane, chlorine, and LPG are some examples.
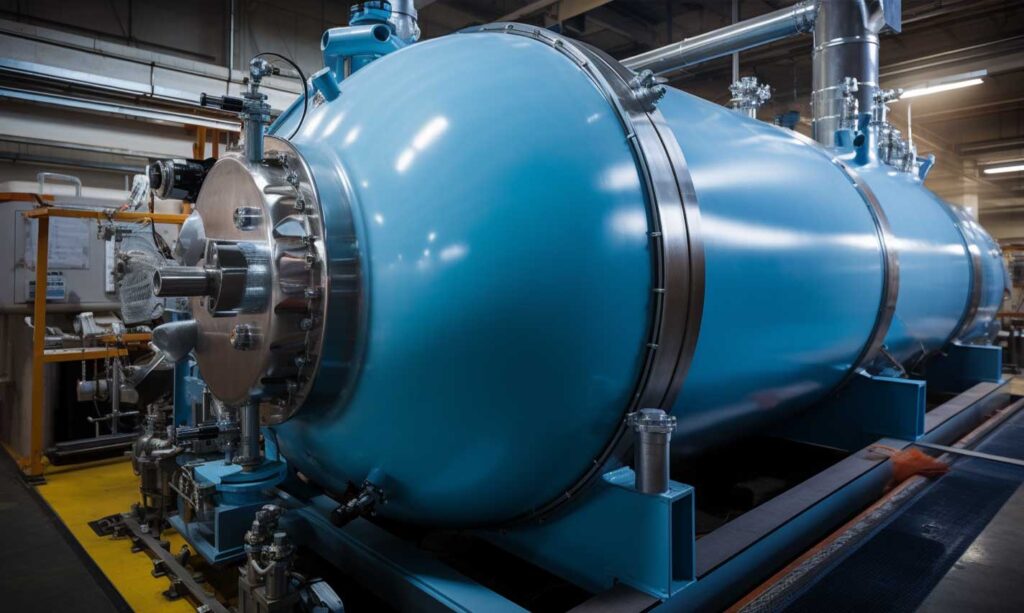
2. Heat Exchangers:
Heat exchangers facilitate heat transfer between two substances without direct contact between the fluids. A collection of metal tubes is present in nearly all pressure vessel heat exchangers. These tubes allow one product to flow through them and another product to flow around them. This leads to the transfer of heat from one product to another. Waste processing plants are one location where you might see this type of pressure vessel in use. In these areas, industries often use heat exchangers to reclaim heat from discarded glass materials.
3. Process Vessels:
Industries integrate these adaptable pressure vessels into production lines, combining various storage units to process diverse products efficiently. Process vessels carry out a variety of operations, such as separation, heating, cooling, purification, blending, and more.
Factories producing colors, pharmaceuticals, and food, as well as petroleum refineries, utilize process vessels in their manufacturing operations.
The thickness of the wall classifies pressure vessels into two main groups. These two kinds of pressure vessels are:

1. Thin Walled Pressure Vessel:
When the thickness of a pressure vessel’s wall is roughly one-tenth or less of its radius, it qualifies as thin-walled. For example, If the radius of a pressure vessel is ‘r’, and if its thickness is ‘t’, then according to the above definition,
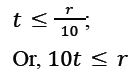
2.Thick Walled Pressure Vessel:
When the thickness of a pressure vessel’s wall exceeds one-tenth or greater of its radius, it qualifies as thick-walled. For example, Suppose a pressure vessel has a radius of “r” and a thickness of “t,” then by the definition given above,

Design specifications, stored product, project site space, and budget all actively shape the size and form of a pressure vessel.
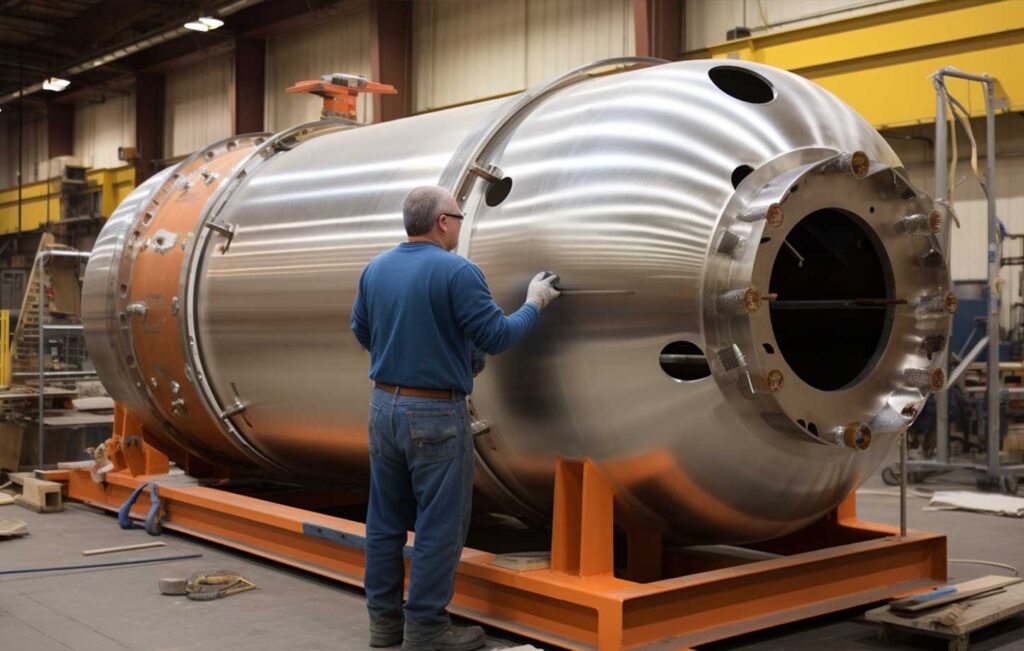
Stresses in Pressure Vessel:
The pressure vessel’s thickness and shape (cylindrical or spherical) determine three types of stress. They are ;
- Tangential or Hoop Stress,σ𝑡
- Radial Stress,σ𝑟
- Longitudinal Stress, σ𝑙
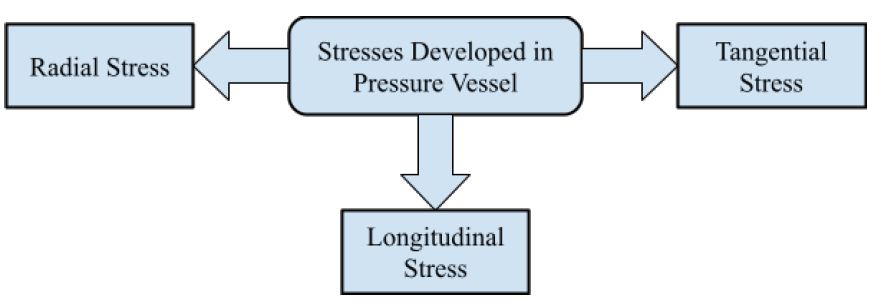
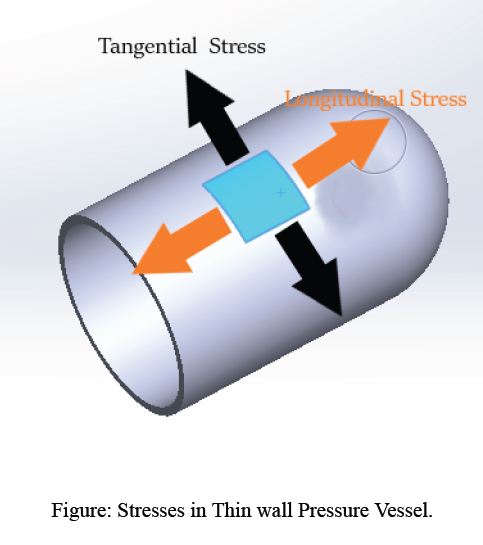
The aforemention image displays a closed thin-walled pressure vessel, with ‘r,’ ‘l,’ and ‘t’ dimensions, under uniform internal pressure ‘p.’ It undergoes solely longitudinal and tangential forces. The Expression for thin wall pressure vessel are given below;
● Tangential or Hoop Stress, σ𝑡= 𝑝𝑟/𝑡; here 𝑝 = uniform pressure exerted inside the Pressure vessel, 𝑟 = radius of the pressure vessel, 𝑡 = Thickness of the vessel.
● Longitudinal or Axial Stress, σ𝑙= 𝑝𝑟/2𝑡;
It follows that Tangential stress (σ𝑡) is equal to twice Longitudinal stress (σ𝑙).

While one can theoretically create pressure vessels in various shapes, manufacturers typically use sections of spheres, cylinders, and cones. A typical design consists of a cylindrical body with heads on the ends. Dished (torispherical) or hemispherical head forms are common. Historically, intricate shapes posed challenges in safety analysis and construction.
Manner of Pressure Vessel Failing:
When a pressure vessel fails, it means that it has sustained enough damage that its strength, stiffness, or functionality are no longer sufficient to satisfy the demands of operation. So how did something like this happen? The failure is actually a process. External mechanical force, medium-temperature conditions, or heat can cause failure by lowering material performance, introducing structural inconsistencies, or diminishing load-bearing capacity.
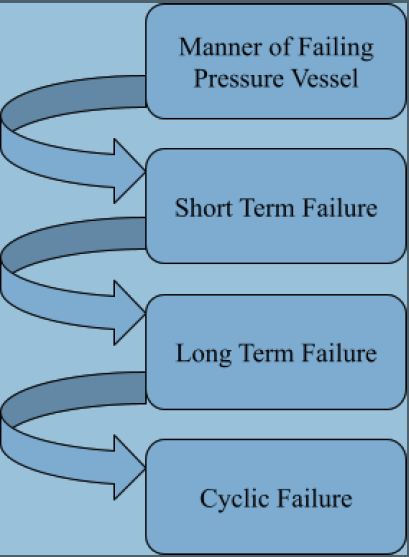
The fundamental principle of a pressure vessel’s failure scenario guides its design method, requiring focused attention in the instructions. Determining the pressure vessel’s potential failure mode should to be the initial stage in the design process. The design of the Class III pressure vessel must also provide risk assessment studies that cover important failure scenarios, risk reduction methods, etc. While evaluating inspection outcomes for pressure vessels, analysts actively consider the failure mode.
Short term failure:
Pressure vessels are made of materials with minimal wall stress and no noticeable plastic deformation. Material brittleness, poor material selection, and flaws in the material itself are the causes of brittle fractures. The stress value approaches the wall’s strength limit when pressure and loads are applied, which leads to fracture. Because of their thin walls, high internal pressure, poor material selection, and poor installation, carbon steel pressure vessels are prone to ductile fracture. Accidents, pollution, and severe environmental harm can result from leakage caused by imperfections in sealing surfaces or loosening nozzles. When compressive stress causes the pressure vessel to abruptly change from its original geometry, instability failure takes place.
Long term failure:
High temperature loads can cause gradual plastic deformation over time in pressure vessels, such as carbon steel tanks, which can lead to failure. Pressure vessel fracture may ensue from this deformation’s notable thickness reduction or bulging deformation. Depending on how resistant the
material is, the pressure vessel’s wall temperature can rise to a level between 25% and 35% of its melting point. Creep instability can result from cumulative creep deformation. The mechanical characteristics of the pressure vessel deteriorate with exposure to corrosive media, such as chemical and electro-chemical deterioration, which ultimately causes the vessel to fail. Consistent cracking, inter-granular, stress, crevice, hydrogen, and bimetallic corrosion are examples of corrosion forms. Cracking that is aided by the environment, such hydrogen-induced cracking and stress-induced cracking, can also happen.
Cyclic failure:
The cyclic failure of a pressure vessel refers to the progressive and repetitive weakening of the vessel’s structural integrity over time, typically as a result of fluctuating internal pressures and stresses. This phenomenon often occurs due to repeated cycles of pressurization and depressurization,
leading to the development of fatigue cracks in the material. These cracks can gradually propagate and compromise the vessel’s strength, eventually culminating in failure. Cyclic loading can be particularly detrimental to pressure vessels, such as those used in industrial applications or energy systems, where they are subjected to varying operational conditions. Mitigating cyclic failure requires careful consideration of material selection, design parameters, and regular inspections to identify potential fatigue issues and prevent catastrophic failures that could pose safety risks.

Fig: Pressure Vessel In Textile Industry
Applications of Pressure vessel in textile industry:
Pressure vessels are used for a variety of reasons in a variety of industries, including textile engineering. Pressure vessels have applications in textile engineering operations such as dyeing, finishing, and other chemical treatments. Here are some common uses of pressure vessels in textile engineering operations:
1. Dyeing Machines:
Pressure vessels are applied in dyeing machines to dye textile fabrics. The pressure forces the dye into the fabric, resulting in a consistent and bright
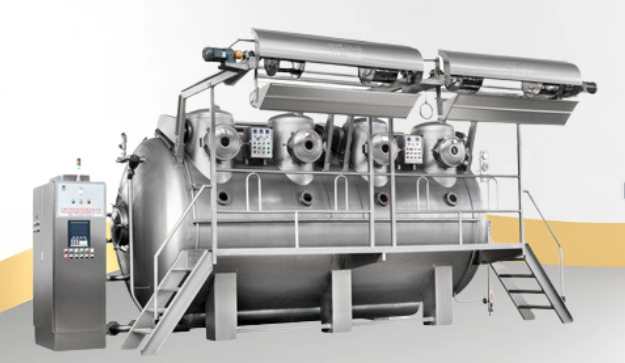
2. Fabric Finishing:
Pressure vessels can be employed in the textile finishing process, when chemicals and finishes are applied to improve qualities like softness, repellent to water, or flame resistance.
3. Textile Printing:
Pressure vessels can be employed in printing procedures to provide pressure to impart designs or patterns onto cloth. This is typical in heat transfer printing processes.
4. Textile Coating:
Pressure vessels are employed in the textile coating process, which involves coating a cloth with a substance to enhance its performance or look. The pressure makes it easier to apply the coating consistently.
5. Chemical treatments for textiles:
Bleaching and desizing are two chemical processes that can be carried out in pressure vessels to speed up the chemical reactions.
6. Textile Bonding and Laminating:
Pressure vessels can be used in the bonding or laminating of textiles, which is the process by which many layers of fabric or other materials are
pressed together to form composite materials.
Efficiently regulating the application of chemicals, dyes, and finishes in textile engineering enhances finished goods’ quality and functionality. Specifically designed vessels resist the required pressure and temperature, prioritizing safety in design, construction, and operation to mitigate potential risks.
Acknowledgements:
- E.P. Popov, Engineering Mechanics of Solids, 1st ed.
- Mechanics of Materials 1. An Introduction to the Mechanics of Elastic and Plastic Deformation of
Solids and Structural Materials (Third ed.) - American Society of Mechanical Engineers (ASME), American Society for Testing and Materials
(ASTM International), National Board of Boiler and Pressure Vessel Inspectors (NBBI)
guidelines.
To learn more on different industrial applications you can check on the following article:
- Reactive Dyeing in textile: mechanism, Classification and steps
- Reactive dyeing: process and method
- Disperse dyes: Properties, Mechanisms, and Application Methods in Textile Industry
- 100% Cotton Knit Fabric Dyeing Process – Exhaust Dyeing Method
- “Stenter Machine: A Textile Finishing Power House”
- How to print Fabrics by using DTF (Direct-To-Film) printing process?
- How to Print a T-Shirt on Direct to Garment (DTG) Printer
- Digital Textile Printing Process – Step By Step Explanation
- Technology Behind the Digital Textile Printing Machine
- “Air Jet Looms: Revolutionizing Textile Production with Speed and Precision”